Simulations Create New Insights Into Pulsars
Explore a new “pulsar in a box” computer simulation that tracks the fate of electrons (blue) and their antimatter kin, positrons (red), as they interact with powerful magnetic and electric fields around a neutron star. Lighter colors indicate higher particle energies. Each particle seen in this visualization actually represents trillions of electrons or positrons. Better knowledge of the particle environment around neutron stars will help astronomers understand how they produce precisely timed radio and gamma-ray pulses.
Credit: NASA’s Goddard Space Flight Center
Music: "Reaching for the Horizon" and "Leaving Earth" from Killer Tracks
Watch this video on the NASA Goddard YouTube channel.
Complete transcript available.
Scientists studying what amounts to a computer-simulated “pulsar in a box” are gaining a more detailed understanding of the complex, high-energy environment around spinning neutron stars, also called pulsars. The model traces the paths of charged particles in magnetic and electric fields near the neutron star, revealing behaviors that may help explain how pulsars emit gamma-ray and radio pulses with ultraprecise timing.
A pulsar is the crushed core of a massive star that exploded as a supernova. The core is so compressed that more mass than the Sun's squeezes into a ball no wider than Manhattan Island in New York City. This process also revs up its rotation and strengthens its magnetic and electric fields.
Various physical processes ensure that most of the particles around a pulsar are either electrons or their antimatter counterparts, positrons. To trace the behavior and energies of these particles, the researchers used a comparatively new type of pulsar model called a “particle in cell” (PIC) simulation.
The PIC technique lets scientists explore the pulsar from first principles, starting with a spinning, magnetized neutron star. The computer code injects electrons and positrons at the pulsar's surface and tracks how they interact with the electric and magnetic fields. It's computationally intensive because the particle motions affect the fields and the fields affect the particles, and everything is moving near the speed of light.
The simulation shows that most of the electrons tend to race outward from the magnetic poles. Some medium-energy electrons scatter wildly, even heading back to the pulsar.
The positrons, on the other hand, mostly flow out at lower latitudes, forming a relatively thin structure called the current sheet. In fact, the highest-energy positrons here — less than 0.1 percent of the total — are capable of producing gamma rays similar to those detected by NASA's Fermi Gamma-ray Space Telescope, which has discovered 216 gamma-ray pulsars.
The simulation ran on the Discover supercomputer at NASA’s Center for Climate Simulation at NASA's Goddard Space Flight Center in Greenbelt, Maryland, and the Pleiades supercomputer at NASA’s Ames Research Center in Silicon Valley, California. The model actually tracks “macroparticles,” each of which represents many trillions of electrons or positrons.
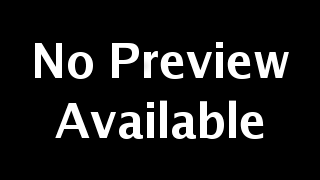
Electrons (blue) and positrons (red) from a computer-simulated pulsar. These particles become accelerated to extreme energies in a pulsar's powerful magnetic and electric fields; lighter tracks show particles with higher energies. Each particle seen here actually represents trillions of electrons or positrons. Better knowledge of the particle environment around neutron stars will help astronomers understand how they behave like cosmic lighthouses, producing precisely timed radio and gamma-ray pulses.
Credit: NASA's Goddard Space Flight Center
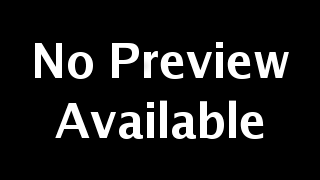
Still image of all particles.
Electrons (blue) and positrons (red) from a computer-simulated pulsar. These particles become accelerated to extreme energies in a pulsar's powerful magnetic and electric fields; lighter tracks show particles with higher energies. Each particle seen here actually represents trillions of electrons or positrons. Better knowledge of the particle environment around neutron stars will help astronomers understand how they behave like cosmic lighthouses, producing precisely timed radio and gamma-ray pulses.
Credit: NASA's Goddard Space Flight Center
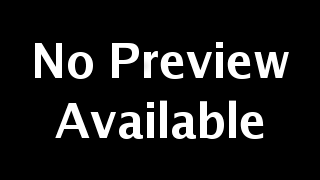
Medium-energy electrons flowing with a pulsar's spinning magnetic field scatter wildly when they near the speed of light. The particles move with the magnetic field, which sweeps back and extends outward as the pulsar spins. Their rotational speed rises with increasing distance, but this can only go on so long because matter can’t travel at the speed of light. The distance where the plasma’s rotational velocity would reach light speed marks the location of a feature astronomers call the light cylinder, a region of abrupt change. As the electrons approach it, they suddenly slow down and many scatter wildly, even back to the pulsar. Others can slip past the light cylinder and out into space. Lighter tracks show particles with higher energies. Each particle seen here actually represents trillions of electrons.
Credit: NASA's Goddard Space Flight Center
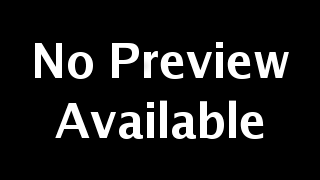
Still image of electron-only simulation with label.
Medium-energy electrons flowing with a pulsar's spinning magnetic field scatter wildly when they near the speed of light. The particles move with the magnetic field, which sweeps back and extends outward as the pulsar spins. Their rotational speed rises with increasing distance, but this can only go on so long because matter can’t travel at the speed of light. The distance where the plasma’s rotational velocity would reach light speed marks the location of a feature astronomers call the light cylinder, a region of abrupt change. As the electrons approach it, they suddenly slow down and many scatter wildly, even back to the pulsar. Others can slip past the light cylinder and out into space.
Credit: NASA's Goddard Space Flight Center
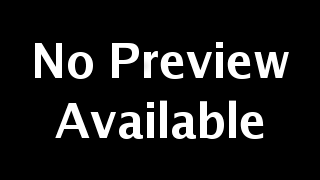
Similar to the still above, but showing the scattering electrons from a different angle. No label.
Credit: NASA's Goddard Space Flight Center
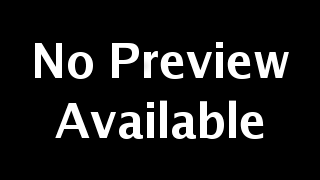
Still image, positrons only, with label.
The pulsar simulation shows that positrons mostly flow out from the surface at lower latitudes. They form a relatively thin structure called the current sheet. Lighter trails indicate greater particle energies. The highest-energy positrons in the simulation represent less than 0.1 percent of the total, but are capable of producing gamma rays similar to those observed, confirming the results of earlier studies. Each particle seen in this visualization actually represents trillions of positrons.
Credit: NASA’s Goddard Space Flight Center
For More Information
Credits
Please give credit for this item to:
NASA's Goddard Space Flight Center. However, individual items should be credited as indicated above.
-
Producer
- Scott Wiessinger (USRA)
-
Visualizer
- Tom Bridgman (Global Science and Technology, Inc.)
-
Science writers
- Francis Reddy (University of Maryland College Park)
- Jeanette Kazmierczak (University of Maryland College Park)
-
Scientists
- Gabriele Brambilla (University of Milan)
- Alice Harding (NASA/GSFC)
-
Narrator
- Scott Wiessinger (USRA)
-
Editor
- Scott Wiessinger (USRA)
Release date
This page was originally published on Wednesday, October 10, 2018.
This page was last updated on Wednesday, May 3, 2023 at 1:46 PM EDT.